Untangling the effect of climatic drivers with space-for-time or manipulation experiments
Summary¶
Human-induced climate change will most likely continue to alter the frequency and magnitude of extreme climatic events in the near-future. It is expected that changes in air temperature (warming) and precipitation regimes will strongly influence soil properties and soil hydrological and biological functioning, while an increase of atmospheric carbon dioxide (CO2) concentration will mainly affect the photosynthetic efficiency of cultivated crops. Soil processes and functioning are of critical importance for the agricultural sector in terms of plant development and food production. Therefore, a range of soil physical (e.g., structure, porosity, water infiltration, and available water), chemical (e.g., soil organic matter), and biological properties (e.g., soil respiration, microbial biomass C and N, microbial diversity, and enzyme activity) have been identified as potential key soil indicators to understand the resilience and adaptative capability of soils to climate change in both short- and long-term. The findings will provide useful information to understand the main biotic and abiotic factors that influence soil and ecosystems health. In this context, field experiments evaluating the adaptative response of different soils to climate change represent a crucial instrument. Generally, in this type of experiments one or more climatic drivers are altered as single- or multi-factor, while all other variables do not change between the different group of treatments. In this synthesis, manipulation field experiments, space-for-time substitution approach, lysimeter, ecotron, and integrated novel approach experiments investigating single- or multi-factorial effects of climate drivers (e.g., warming and extended drought) and elevated atmospheric CO2 on soil properties and functioning were considered. Particularly, manipulation experiments, ecotron and lysimeter research monitor one or more processes and properties in the soil-plant system, following artificial alteration of either precipitation, temperature or partial pressure of pCO2 regimes. Conversely, space-for-time substitution soil properties and processes are studied at large spatial scales encompassing a wide range of climates. This synthesis resumes the state of the art of knowledge and knowledge gaps on impacts of changing climatic drivers on soil properties and functioning and adaptation of soil variables to climate change. This report aims at synthesizing and integrating scientific information and evidence on existing studies in order to qualitatively assess the individual or combined effects of climate drivers on soil properties and functioning, mainly related to water infiltration and availability, nutrients availability, soil organic carbon, enzyme activity, and microbial biomass.
The peer-reviewed literature that we have selected to compile this synthesis has been published over 14-years, from 2008 to 2021. The interest of scientific community to better understand the response of terrestrial ecosystems and soils to climate change has increased over the past decade. A total of 39 unique peer-reviewed literature articles were included in this report. Most of the peer-reviewed literature, selected for this report, refers to original research paper, followed by synthesis and review, and meta-analysis. Particularly, the selected peer-reviewed literature focused on studies and observations referring to different scales from national to global, with a greater concentration of data collected in field experiments carried out in Europe, worldwide, or at local level in one Country outside Europe.
Most authors agree that field manipulation experiments are powerful tools to understand the resilience and adaptative response of soils to face climate change because they allow to clarify the cause-and-effect relationships in both short- and long-term. Soil response to climatic extremes such as drought events is currently studied worldwide in a growing number of field experiments that predominantly use rain-out shelters. The space-for-time substitution approach is an indirect method used for investigating the effects of climatic variation on soil properties and functioning. Particularly, climatic time-series collected in a multiple-site gradient area allow to simulate climate change scenarios current climate to altered temperature and precipitation regimes such as warming, droughts, and floods. Lysimeter experiments measure water budget, C and N cycling in the unsaturated and saturated zone of the entire soil profile. Frequently, they are used to quantify the impacts of climate variables on the soil–plant system. Econtron experiments are designed to realistically simulate above- and below-ground environmental conditions by automatically measuring ecosystem processes.
It is well-known that altered precipitation conditions affects both soil abiotic (e.g., water availability, infiltration, and runoff) and biotic (e.g., microbial community and enzyme activity) processes. Regarding abiotic process, long-term increase of water inputs, used to simulate altered precipitation regime, affects soil hydraulic property, by substantially reducing infiltration rates and influencing water retention, especially during dry summer, that can negatively affect microbial abundance and activity. Considering biotic processes, also below-ground C and N cycling are highly influenced by changes in precipitation regimes. Particularly, an increase in precipitation regime may accelerate soil organic matter decomposition, while drought events may reduce the decomposition due to the increased physical protection of soil organic matter. Conversely, bacterial, and fungal communities are relatively resistant to decreased precipitation. Some manipulation experiments, investigating the combined effects of climatic drivers and enhanced atmospheric CO2 concentration on soil properties, highlight that they directly influence soil water and microbial processes linked to soil organic matter decomposition.
This synthesis covers more than 30 soil variables, such as soil GHG emission, soil water content and hydraulic properties, C and N cycling, meso-fauna (e.g., earthworms and nematodes), microbial community (e.g., fungal and bacteria), and enzyme activity. On the other hand, 7 and 15 publications specifically investigated the individual effect of altered temperature and precipitation, respectively, while the combined effect of climate variables (i.e., “CO2 + T”, “CO2 + P + T”, or “P + T”) were investigated in 16 publications.
In general, this synthesis provides a clear overview of the current knowledge on the adaptative response of key soil properties and functioning to climate change based on field approaches. The report advanced our understanding on the impact of climate drivers and enhanced CO2 concentration on soil properties and functioning mainly related to grassland land use. Therefore, more insights need to be obtained for other land use and soil management practices. This is of crucial importance to help us understand the complex mechanisms underlying the responses of soil system to climate change that are still not completely known.
Introduction¶
Aim of this synthesis¶
Understanding the direct impact of climate change on soil properties (e.g., soil structure, porosity, and organic matter stock) and functions (e.g., water infiltration and storage) are crucial for identifying research needs to advise practitioners and policymakers. Nevertheless, climate change is a slow process, many of whose effects are only seen at very long time-scales. Adapting to these changes requires knowledge of ecosystem functioning in the expected novel environments through field experimentation and modelling.
To date, many manipulation experiments have been carried out in which one or more processes and properties in the soil-plant system are monitored following artificial alteration of either precipitation, temperature or partial pressure of carbon dioxide (pCO2) regimes. Space-for-time substitution (SFT) is an alternative approach, whereby properties and processes are studied at very large spatial scales encompassing a wide range of climates. Also, papers adopting a gradient design approach will be examined. Finally, we assessed ecotron and lysimeter research results of studies investigating the impacts of climatic drivers (altered temperature and precipitation regimes) and pCO2 (i.e., enhanced concentration) on soil hydrological and biological functioning. Apart from synthetizing knowledge on direct impacts of climatic drivers on soil properties and functions, this chapter also highlights challenges and opportunities of the different types of experiments analysed in this study.
Background¶
Increasing greenhouse gas (GHG) emissions are expected to raise global mean temperature by the end of this century (IPCC, 2021). Temperature and precipitation are key climate drivers that affect ecosystem processes Wu , 2011 , with strong ecological implications Kreyling , 2017. Thus, climate change is expected to drive soil and ecosystem variables by directly altering the equilibria of soil processes and by indirectly influencing soil physical structure and biota. Particularly, changes of air temperature (warming) and shifts in precipitation regimes (e.g., amount, intensity, and frequency) have vigorous impacts on soil biogeochemical and hydrological cycles Gelybó , 2018, while the increase of atmospheric carbon dioxide (CO2) levels mainly affects the crops photosynthetic efficiency Tubiello , 2007. The specificity and magnitude of external abiotic and biotic impacts strongly influence terrestrial carbon (C) balance (Wu (2011) and the adaptability of soils Gelybó , 2018Tóth, 2008 . Soil types, land use, and management are differently sensitive to climate change depending on their properties and functioning. Understanding the sensitivity of terrestrial C balance to climate change is a high priority, because of the potential of soils for C storage Cox , 2000. It is well-known that soils represent the third largest reservoir of C on the Earth - after oceans and carbonate rocks – and plays a key role in terrestrial ecosystems Zhang , 2008. It is estimated that soil carbon stocks account for 2500 Gt, which include about 1550 Gt of soil organic carbon (SOC) and 950 Gt of soil inorganic carbon Lal , 2007. In European regions, soil types are highly vulnerable to SOC loss, with western and eastern parts of the EU considered at high risk, while soils in southern parts have complex pattern of regions with low and high risks of SOC loss Stolbovoy , 2008. These changes are also influenced by other abiotic factors such as soil texture and water content. As stated by Ćirić (2017), heavy textured, deep (Vertisols), and calcareous soils (Chernozems), generally rich in SOC and well-structured are less sensitive to weather extremes compared to the shallow (Umbrisols) soils, with coarse texture (Arenosols) and poorly aggregated, generally characterized by low SOC (Fluvisols, Cambisols) and affected by water (Gleysols, Gleyic Chernozems). A range of soil physical, chemical and biological properties are highlighted as potential key soil indicators in relation to climate change. Determining response of soils to climate change based on different soil types and land use management provides useful information on potential soil degradation in the future and might be used as a guideline for soil protection Ćirić , 2017. In this context, soil covered by natural vegetation are lesser affected by projected climate change compared to the soils under intensive agricultural management. Thus, land use and management changes such as conversion from arable to grasslands or increasing crop diversity are expected to limit soil degradation. Figure 1 shows a schematic representation of the potential links among climate change, agricultural soil management, and soil properties.
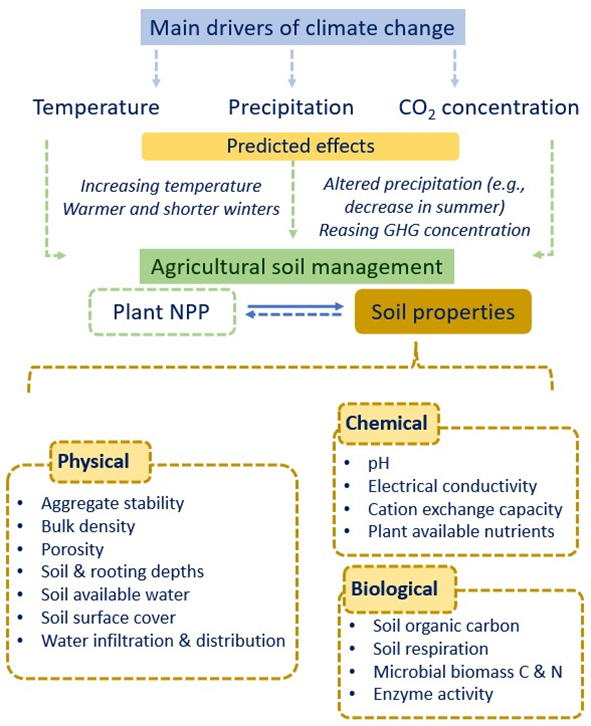
Schematic representation of the potential links among climate change, agricultural soil management, and soil properties (adapted from Nuttall (2007) and Allen (2011)).
A range of soil properties and functions identifies the status of soils and their relevance to climate change drivers (Table 1). The soil physical properties listed in Table 1 provide information related to soil structure, porosity, water infiltration, and available water, while the soil chemical and biological properties mainly affected by climate change are related to SOM, soil respiration (Rs), microbial biomass C and N (MBC and MBN), microbial diversity, and enzyme activity. The frequency of their inclusion within a minimum data set were also provided. The selection of these key indicators can be useful to assess the effect of climate change on soil properties and functions, as well as the adaptive capability of soils and their resilience to climate change in both short- and long-term.
Summary of key soil property and function measured or estimated from pedotransfer functions, that are directly or indirectly affected by climate change (adapted from Allen (2011)).
Compartment | Soil property | Soil function | Relevance to climate change | Note |
---|---|---|---|---|
Physical | Structure |
| High |
It gives information on:
|
Physical | Porosity |
| High |
It gives information on:
|
Physical | Infiltration |
| High |
It influences:
|
Physical | Soil/plant available water |
| High |
It gives information on:
|
Chemical | SOM (light fraction or macro-organic matter) |
| High |
It gives information on:
|
Biological | Soil respiration and microbial biomass C and N |
| High |
It gives information on:
|
Biological | Microbial diversity and enzyme activity |
| High |
It gives information on:
|
Aggregate stability and porosity are determined by soil structure and are related to the resistance of soil aggregates to external drivers such as high intensity precipitation and soil tillage management. Since these processes are related to other soil physical (e.g., water infiltration and storage), chemical, and biological properties (e.g., SOC and microbial activity), they are considered useful indicators to identify the most suitable soil adaptation strategies, especially in those areas where high and intense precipitation are likely to occur Allen , 2011.
Infiltration refers to the rate at which water enters the soil surface and moves through soil depth Allen , 2011. Soil water infiltration is controlled by other soil physical properties (e.g., porosity, field capacity, macropore flow, and texture) and it is considered an important soil property that regulates leaching, runoff, and water availability for plant growth Franzluebbers, 2002Jarvis, 2007. In this context, water availability and water distribution into soil are key processes highly influenced by extreme events (e.g., high intensity precipitation or drought) and soil management strategies Allen , 2011. For instance, the adoption of management strategies that maintain or even enhance water infiltration and storage in soil (e.g., conservation tillage, planting of cover crops, and incorporation of organic matter) may help to mitigate the negative impacts of extreme events (e.g., severe precipitation or drought), limiting the risk of erosion Lal , 2007Sanchis , 2008.
It has been widely acknowledged that SOM is one of the most complex and heterogeneous components of soils because it is composed by an extensive range of living and non-living materials. SOM supports most of the soil processes Franzluebbers, 2002, associated with soil fertility, physical stability, and biological activities Karmakar , 2016. It is influenced by external drivers (e.g., climate and land management) and it can act both as a source and a sink of C in terrestrial ecosystems, thus potentially mitigating or accelerating the greenhouse gas (GHG) emission effects Karmakar , 2016. In this context, a decrease of SOM can negatively influence soil quality and health, by reducing soil structure, water holding capacity, and biodiversity, and increasing the risk of soil erosion and compaction Allen , 2011Karmakar , 2016 .
Rs soil microbial biomass, and enzyme activity are positively correlated with SOM content, and they can easily detect short-term variations in soil processes. Rs is a key biological indicator for soil health, and it can be determined as either CO2 production or O2 consumption (e.g., soil or basal respiration). It is widely acknowledged that Rs, soil microbial biomass, and enzyme activity are highly responsive to changes in temperature and seasonal precipitation regimes, which are predicted to change according to global and regional climate models Allen , 2011.
Main objectives¶
This report aims at synthesizing and integrating scientific information and evidence from peer-reviewed literature on existing field manipulated experiments and SFT substitution studies in order to provide a general overview of the responses of (agricultural) soils to climate change by qualitatively assessing the individual or combined effects of enhanced atmospheric CO2 concentration and climate drivers such as warming, droughts, and floods on soil properties (physical, chemical, and biological) and functioning. Particularly, aspects related to soil available water, water infiltration, plant available nutrients, soil organic carbon (SOC), microbial biomass and diversity, and enzyme activity are examined.
The specific objectives of the report are to:
- Identify the most important knowledge and knowledge gaps on the relationship between predictive climate change and adaptation strategies of agricultural soils. Here the knowledge gap refers to insights not yet or fully available, generally caused by a lack of essential data.
- Provide recommendations and propose future research directions to integrate manipulation field experiments, SFT substitution approach, lysimeter studies, and ecotron experiments, using a multidisciplinary approach.
Methodology¶
In February/March 2021, a brainstorming exercise was carried out to identify potential keywords related to climate and soil. In agreement with the CLIMASOMA project consortium, the most important keywords were identified and selected to be used as search string for collecting relevant published literature. For climate drivers, the selected keywords mainly focused on air temperature, drought, flood, change in precipitation intensity, and climate aggressiveness, while for soil properties and functioning to soil organic matter (SOM), SOC, microbial activity, water storage, and water infiltration. This report was compiled by CREA (Italy) in collaboration with EV-ILVO (Flanders, Belgium) and SLU (Sweden). Figure 2 shows the general overview of plan activities used to identify keywords and to collect, process, and extract relevant information from the selected peer-reviewed literature, useful to compile this synthesis report.
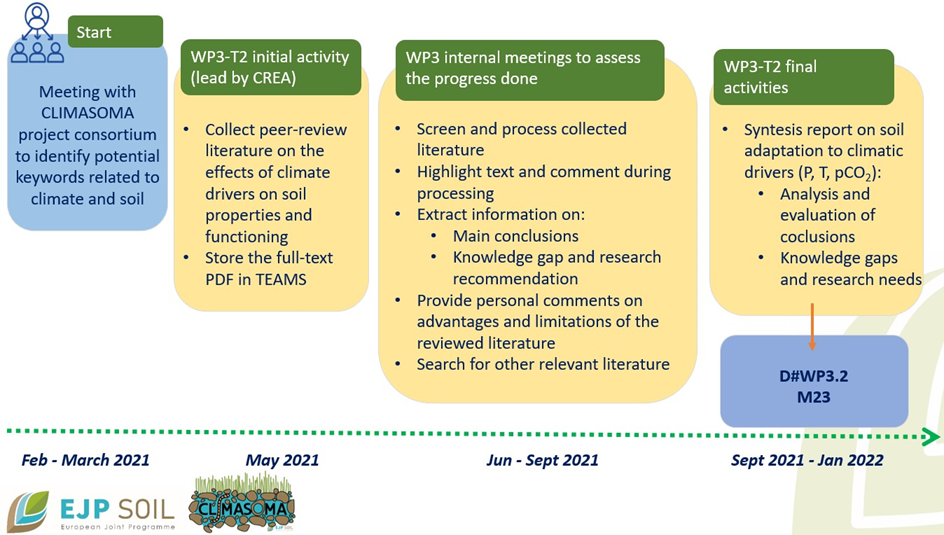
Overview of plan activities used to identify keywords and to collect, process, and extract relevant information from peer-reviewed literature, useful to compile the synthesis report.
Literature review methodology¶
From May to October 2021, we conducted a literature search to collect the published peer-reviewed scientific articles about the individual or combined effects of climate drivers (i.e., decreased and/or increased temperature and precipitation) and enhanced CO2 concentration on soil chemical, biological, and hydrological functioning. Only scientific peer-reviewed literature written in English and published until October 2021 was included in our analysis. Here, publications different from scientific articles such as books, book chapters, theses, congress proceedings, and other grey literature written in English or in other languages of the CLIMASOMA project consortium were excluded because the accessibility and efforts to collect them overpassed our ability to manage this information. The engines consulted to search the literature were Scopus, ISI Web of Science, and Google Scholar. For qualitatively assessing the single- or multiple-factor effects of climate change on soil properties and functioning, the following combination of search terms were used:
- Temperature: “altered temperature”, OR “increased temperature”, OR “warming”, OR “climate change”
- Precipitation: “altered precipitation”, OR “precipitation addition/removal”, OR “increased/decreased precipitation”, OR “rainfall addition/removal”, OR “increased/decreased rainfall”, OR “drought”, OR “water addition/reduction”
- Atmospheric CO2 concentration: “elevated CO2”, OR “increased CO2”, OR “CO2 fertilization effects”
- Soil linked water and physical terms: “available water”, OR “water retention”, OR “hydraulic conductivity”, OR “moisture”, OR “infiltration”, OR “aggregate stability”, OR “aggregation”
- Soil linked chemical and biological terms: “organic matter”, OR “degradation, OR “stress”, OR “respiration” OR “CO2” OR “carbon” OR “nitro” OR “N2O” OR “CH4” OR “GHG”, OR “microb” OR “enzyme” OR “bacteria” OR “fungi”, OR “faunal”
- Ecosystem type and land use system: “grassland”, OR “cropland”, OR “meadow”, OR “peatland”, OR “tundra”, OR “agricultural system”, OR “mixed”, NOT “urban”
- Type of field experiments: “manipul”, OR “space-for-time substitution approach”, OR “gradient design”, OR “lysimeter”, OR “ecotron”.
Generally, the dataset of the selected literature covered a limited range of land use, ecosystems, types, and biomes, mostly oriented to grassland or semi-natural systems. Specifically, only few studies were focused on agricultural land and cropping systems (e.g., Poll (2013)). Therefore, to enlarge our understanding on the effects of climate drivers on soil variables, meta-analysis, synthesis and review, encompassing a wide range of soil characteristics such as soil C and N cycling, microbial communities, enzyme activity, and soil physical properties were also considered.
For inclusion into the synthesis, the original research papers, meta-analysis, synthesis and review had to meet the following criteria:
- They had to refer to field studies where the magnitude of climate drivers was manipulated or a SFT approach was used in order to compare the effects of current and future climate on the soil response variable(s);
- they needed to study the effect of at least one climate variable, including atmospheric CO2 concentration increase, temperature or precipitation changes;
- they needed to contain at least one response variable from the following list: microbial community, C and N cycling, SOC change, soil respiration, water storage;
- they had to be relevant for European agriculture and environmental zones.
Applying the above-mentioned criteria, 39 studies were found suitable for this synthesis.
Results and discussion¶
A total of 39 unique peer-reviewed literature articles (Table 2) were included in this report. Most of the peer-reviewed literature, selected and analysed in this report, refers to original research paper (76.9%), followed by synthesis and review (20.5%), and meta-analysis (2.5%), as reported in Table 2.
Type and number of experiments | Number |
---|---|
Original research paper | 30 |
Synthesis and review | 8 |
Meta-analysis | 1 |
The peer-reviewed literature that we have selected to compile this synthesis has been published over 14-years, from 2008 to 2021. Figure 3 shows the number of peer-reviewed scientific articles published per year that has been included in this report. A total of 8 selected papers (20.5%) have been published in the first period (from 2008 to 2014), while the other 79.5% in the second period (from 2015 to 2021). The year 2016 presented a peak (n=6), 2020 reached the highest number of publications (n=10), and 2021 had another peak (n=5), although the data for this year was incomplete as our search ended in October 2021. This is explained by the fact that the publication of the selected peer-reviewed literature focused on the impact of changing climatic drivers on soil processes and functioning was mainly published after 2015. The interest of scientific community to better understand the response of terrestrial ecosystems and soils to climate change has increased over the past decade.
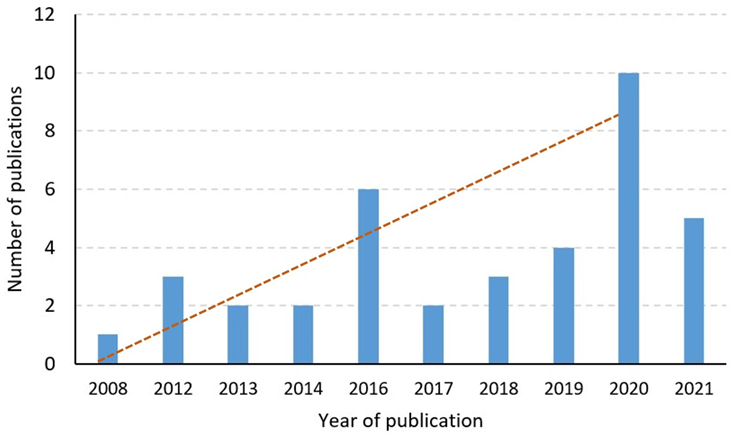
Overview of the selected digital peer-reviewed literature published until October 2021 and grouped per publication year. There is an increasing trend in the number of publications.
Although this synthesis mainly focused on studies carried out in grassland and cropland, we have also selected peer-reviewed literature that considered other ecosystem types to have a more comprehensive framework of the implication of climate drivers on soil properties and functioning. Therefore, 4 types of land use were included in this report (Table 3). Cropland and grassland were investigated in most of the experimental studies (56%), followed by mixed heterogeneous areas (36%), and meadow/prairie (8%).
Type of land use, reporting the number of records as found in peer-reviewed selected literature.
Type of land use | Number |
Cropland | 12 |
Grassland | 16 |
Meadow/Prairie | 4 |
Mixed heterogeneous areas* | 18 |
* Mixed heterogeneous areas include heathland (5), forest (8), peatland (2), steppe (2), and tundra (2) |
A further classification of the selected peer-reviewed literature was done considering the geographic coverage of the experimental studied. Here, a national publication refers to a study carried out in one or more locations of a specific country, a regional publication describes research investigating effects for part of Europe (at least two Countries), and global publication refers to meta-analyses or synthesis and reviews carried out in some countries worldwide. Particularly, the selected peer-reviewed literature focused on studies and observations referring to different scales from national to global, with a greater concentration of data collected in field experiments carried out in Europe (47.4%), worldwide (26.3%), or at local level in one Country outside Europe (26.3%), especially in the United States (USA; 50% of the local studies outside Europe), as shown in Table 4.
Approaches to assess adaptation and response of soil properties to climate drivers¶
Extreme climatic events are predicted to increase in frequency and magnitude in the near-future, with strong implications for soils and ecosystems Kreyling , 2017Rustad, 2008. As stated in the most recent literature on this topic, the ecosystem climate change experiments represent a crucial instrument to study the adaptive response of ecosystems to climate change Roy , 2021Vanderkelen , 2020. Generally, in this type of experiments, different climatic variables are altered (e.g., temperature, precipitation, and CO2 concentration) as single- or multi-factor. Generally, in these experiments one or more climate drivers are altered, while all other variables do no change and are held equal between the different group of treatments.
In this synthesis, field experimental studies (i.e., manipulation, lysimeter, and ecotron experiments) or SFT substitution approach that investigate the single- or the multi-factorial effects of climate drivers (e.g., warming and extended drought) and elevated atmospheric CO2 on soil properties and functioning were considered. Particularly, we focused on determining how properties of agricultural and grassland soils can be altered by single or simultaneous climate driver changes and how such soils can adapt to these climatic changes.
Table 5 summarizes the type and number of experiments that were included in the selected peer-reviewed literature. The most widespread type of experiment used to assess the single- or multi-factor effects of climate drivers on soil properties is represented by manipulation field experiment (30 input rows, 73.2%), followed by SFT substitution approach (5 input rows, 12.2%). Conversely, the lysimeter, ecotron, and integrated novel approach experiments were less adopted in the peer-reviewed literature we have selected to compile this synthesis (2 input rows each, covering together 14.6% of the total evaluated experiments).
Manipulation experiment¶
Most authors agree that field manipulation experiments are powerful tools to understand ecological processes Smith , 2014Kreyling , 2017 and resilience of soils in the face of climate change (e.g., Breitkreuz (2021), Alatalo (2017), Carey (2016)). As stated by Rustad (2008), experimental manipulations allow to better understand the the cause-and-effect relationships and the responses of ecosystems to single or multiple elements of global in both short- and long-term. Soil response to climatic extremes such as drought events is currently studied worldwide in a growing number of field experiments that predominantly use rain-out shelters (e.g., Kundel (2018)).
Experimental manipulations can help us to understand the adaptative response of ecosystem or soil variables to single- or multiple-factor climate drivers Rustad, 2008. However, results from short-term manipulation experiments may be transient, thus it is important setup long-term ecosystem manipulation experiments validate this concern and incorporate the findings into soil-crop and ecosystem models.
Space-for-time substitution approach¶
SFT substitution approach is commonly used in ecology and terrestrial systems to estimate implications for ecosystem functions. It refers to the extrapolation of a temporal trend from a series of different-aged samples Pickett, 1989. The SFT is a type of “natural experiment” Walker , 2010 that mimics a forecasted change over time, where spatially separated zones along a natural climatic gradient Fleischer & Sternberg, 2006 serve as proxies for predicting temperature and/or precipitation changes Rustad, 2008. The SFT approach is an indirect method used for investigating and understanding the effects of climatic variation on ecosystems Koch (1995). To better quantify these effects on soil properties and functioning, specific environmental factors such as altitude and topography must be also considered in the same gradient framework Dunne , 2003Dunne , 2004. Particularly, climatic time-series collected in a multiple-site gradient area allow to simulate climate change scenarios in such area ranging from no climate change (current climate) to altered temperature (warming) and/or precipitation (droughts and floods).
Lysimeter experiment¶
Weighable lysimeters are vessels filled with disturbed or undisturbed soil volumes, isolated from the surrounding field conditions. They are frequently used tools to measure water and matter fluxes in the unsaturated and saturated zone of the entire soil profile (up to several meters deep) and can provide accurate and precise observations up to field scale. Frequently, they are used to quantify the impacts of climate variables on the soil–plant system such as the influence of higher soil temperature and CO2 concentration on water budget C and N cycling. A more detailed description of lysimeters is reported in Groh , 2016Groh , 2020.
Ecotron experiment¶
Ecotron is an experimental facility including a set of meso- macro-cosmos (i.e., replicated enclosures) that is designed to host ecosystems samples and allow realistic simulations of aboveground and belowground environmental conditions by automatically measuring ecosystem processes. Thus, it provides continuous information on ecosystem functioning (e.g., fluxes of energy and matter). Ecotrons used enclosures composed by two parts: a lysimeter for the soil and an aerial compartment around the canopy. A more detailed description of ecotrons is reported in Granjou & Walker (2016), Roy (2021), Schmidt (2021).
Effects of climate drivers and enhanced CO2 concentration on soil properties¶
Table 6 lists the main soil variables that were investigated in study described in the selected peer-reviewed literature as well as the number of studies grouped per type of individual or combined climate variables (i.e., altered temperature, precipitation, and enhanced CO2 concentration). This synthesis covers more than 30 soil variables, encompassing a wide range of soil characteristics such as soil GHG emission, soil water content and hydraulic properties, C and N cycling, meso-fauna (e.g., earthworms and nematodes), microbial community (e.g., fungal and bacteria), and enzyme activity. Regarding climate variables, no publications investigated the single effect of enhanced CO2 on soil properties and functioning, while 7 and 15 publications specifically investigated the individual effect of altered temperature and precipitation, respectively. On the other hand, the combined effect of climate variables (i.e., “CO2 + T”, “CO2 + P + T”, or “P + T”) were investigated in 16 publications (Table 6).
List of main soil variables investigated in the selected studies to assess the effects of individual and combined climate drivers (i.e., decreased and/or increased temperature and precipitation) and CO2 concentration on soil.
Soil variable | T | P | CO2 | Combined effects | Reference |
Rs, SOC, GHG, MBC, MBN, microbial community, enzyme activity, Total and mineral N, belowground biomass |
| x |
|
| |
Microbial community |
| x |
|
| |
Physical (e.g., structure, texture, compactness, pore size and pore distribution) and biological (e.g., microbial and faunal biomass and community, rooting depth and root distribution) |
| x |
|
| |
Hydraulic properties |
| x |
|
| |
Moisture, hydrophobicity (water repellence) |
| x |
|
| |
Water storage, crop water use efficiency |
| x |
|
| |
Moisture, temperature |
| x |
|
| |
Near-saturated hydraulic conductivity, infiltration |
| x |
|
| |
Water content, microbial community, C, N |
| x |
|
| |
Water content, evapotranspiration |
| x |
|
| |
Water content, evapotranspiration |
| x |
|
| |
Moisture |
| x |
|
| |
C, N |
| x |
|
| |
Water, Rs |
| x |
|
| |
C, N, moisture, and mites | x |
|
|
| |
Microbial community composition | x |
|
|
| |
Rs | x |
|
|
| |
Rs, GHG, moisture, temperature | x |
|
|
| |
Gross N mineralization, nitrification, abundance of ammonia-oxidizing archaea and bacteria, and other supporting soil parameters including gravimetric water content, concentrations of soil ammonium and nitrate, dissolved organic nitrogen and dissolved organic carbon | x |
|
|
| |
Water holding capacity, SOM | x |
|
|
| |
Belowground biomass, C, water content, MBC, MBN, bacterial and fungal biomass | x |
|
|
| |
Belowground biomass, Rs, C storage, and nutrient cycling |
|
|
| x | |
Soil C |
|
|
| x | |
Water balance components |
|
|
| x | |
Dissolved inorganic N, mineral N, N2O emission, C, N, evapotranspiration |
|
|
| x | Giraud , 2021 |
Water content |
|
|
| x | |
Moisture, CH4 and CO2 emission |
|
|
|
| |
Moisture |
|
|
| x | |
MBC, MBN, microbial community composition |
|
|
| x | |
Aggregate dynamics, SOM, CO2 flux |
|
|
| x | |
Microbial biomass, nematode densities |
|
|
| x | |
MBC, CO2 flux, temperature, moisture |
|
|
| x | |
GHG emission |
|
|
| x | |
Rs, N mineralization |
|
|
| x | |
Earthworm community, moisture, pH |
|
|
| x | |
Moisture, Rs, |
|
|
| x | |
Water content, water tension, electrical conductivity, temperature |
|
|
| x | |
C, N, MBC |
|
|
| x | |
Total | 7 | 15 | 0 | 16 |
|
Note for experimental factors: T (altered temperature), P (altered precipitation), and enhanced CO2 concentration (CO2). |
From each selected peer-reviewed literature, the most relevant information such as type of experiment (i.e., manipulation, SFT, econtron, and integrated methods), experimental factors (i.e., climate variables, individual: “P” and “T”; combined: “CO2 + T”, “CO2 + P + T”, or “P + T”), spatial level of research (national, European, global) and main conclusions was extracted and summarised in Table 7. Since two studies Forstner (2021) and Rahmati (2020) investigated two different approaches, a total of 40 records were listed in Table 7.
List of type of field experiments, investigated factor(s), and main conclusions from the selected studies, examining the effects of individual and combined climate drivers (i.e., decreased and/or increased temperature and precipitation) and enhanced CO2 concentration on soil functioning.
N. | Exp. type | Exp. factor(s) | Author(s) | Level | Land use | Main conclusions |
1 | Manipulation | P | global | grassland |
| |
2 | Manipulation | P | global | grassland |
| |
3 | Manipulation | P | global | grassland, cropland |
| |
4 | Manipulation | P | local | prairie |
| |
5 | Manipulation | P | local | forest |
| |
6 | Manipulation | P | local | tundra |
| |
7 | Manipulation | P | local | peatland |
| |
8 | Manipulation | P | local | grassland |
| |
9 | Integrated novel approach | P | local | cropland |
| |
10 | Manipulation | P | global | grassland |
| |
11 | Manipulation | P | local | grassland |
| |
12 | Manipulation | P | local | heatland |
| |
13 | Manipulation | P | global | grassland |
| |
14 | Manipulation | T | local | meadow |
| |
15 | Manipulation | T | local | cropland |
| |
16 | Manipulation | T | global | cropland, forest, grassland, heathland, meadow, peatland, tundra |
| |
17 | Manipulation | T | local | forest |
| |
18 | Manipulation | T | local | steppe |
| |
19 | Manipulation | CO2 + T | global | grassland, forest |
| |
20 | Manipulation | CO2 + T | EU | grassland | • Manipulation: higher CO2 concentration on ETa largely compensated by higher temperatures. | |
21 | Manipulation | CO2 + T | local | cropland |
| |
22 | Manipulation | CO2 + P + T | local | grassland, heatland |
| |
23 | Manipulation | CO2 + P + T | global | grassland, forest | Retrospective summary of several key publications on experimental manipulations field experiments and suggestions to:
| |
24 | Manipulation | CO2 + P + T | local | cropland |
| |
25 | Manipulation | P + T | local | forest |
| |
26 | Manipulation | P + T | local | forest |
| |
27 | Manipulation | P + T | local | grassland |
| |
28 | Manipulation | P + T | local | forest |
| |
29 | Manipulation | P + T | local | steppe |
| |
30 | SFT | P | local | cropland |
| |
31 | SFT | P | local | grassland |
| |
32 | SFT | T | local | grassland, cropland, forest |
| |
33 | SFT | CO2 + T | EU | grassland |
| |
34 | SFT | P + T | Giraud , 20211 | local | cropland |
|
35 | Lysimeter | P + T | local | cropland |
| |
36§ | Lysimeter | P + T | local | grassland |
| |
37 | Ecotron | T | local | prairie |
| |
38 | Ecotron | P + T | local | heatland |
| |
39 | Integrated novel approach | P | local | cropland |
| |
40 | Integrated novel approach | CO2 + P + T | global | cropland, grassland |
| |
Type of peer-reviewed literature: 1Original research paper, 2Synthesis and review, 3Meta-analysis. Experimental factors: enhanced CO2 concentration (CO2), P (altered precipitation), T (altered temperature). §Lysimeter network for observing soil processes and plant diversity as affected by climate change |
In precipitation manipulated field experiments the effect of a treatment is generally assessed compared to a control or a reference condition Beier , 2012. Many authors agree that altered precipitation conditions affects both soil abiotic (e.g., water availability, infiltration, and runoff) and biotic (e.g., microbial community and enzyme activity) processes Alster , 2013Caplan , 2019Wang , 2021. For instance, an increase in precipitation regime may accelerate SOM decomposition and plant growth due to higher water availability in soil compared to unchanged precipitation condition, while drought events may reduce the decomposition due to the increased physical protection of SOM Vasconcelos , 2004Wang , 2021Wu , 2011. These behaviours may have direct consequences for soil C pools Fröberg , 2008. Abbasi (2020) conducted a comparative analysis of 16 peer-reviewed meta-analyses, exclusively oriented on field studies (covering different land use, mainly based on grassland) where the magnitude of precipitation was manipulated. Particularly, the authors deeply evaluate the effects of altered precipitation on different soil response variables (n=42), covering a wide range of soil processes (e.g., GHG fluxes, C and N cycling, microbial community, and enzyme activities). Much attention has been paid to fluxes and pools. The authors found strong agreement among the examined studies that belowground C and N cycling are highly influenced by changes in precipitation regimes. Both cycles speed up under increased precipitation and slow down under decreased precipitation. Conversely, bacterial and fungal communities are relatively resistant to decreased precipitation.
It is well-known that precipitation regimes are expected to change at accelerating rates globally. Thus, changes in soil structure could occur over broad regions, thus altering water infiltration and storage in many terrestrial ecosystems. In this context, the recent paper published by Caplan (2019) specifically focused on the decadal-scale shifts in soil hydraulic properties as induced by altered precipitation. In a typical prairie of Kansas (US) managed over 25-year under a precipitation manipulation experiment, the authors found that soil with a 35% increase in water inputs substantially reduced infiltration rates and modestly increased water retention. The authors suggested that the shifts reduced shrink-swell cycles and it can be catalysed by greater pore blockage by plant roots.
In a manipulation field experiment setup in a temperate agricultural ecosystem in Germany, Poll (2013) tested the combined effects of altered temperature (warming) and precipitation (amount and frequency) on soil properties. Interestingly, the authors found a negative effect of elevated soil temperature on soil moisture, which could be explained by increasing evapotranspiration (Eta). This might induce water limitation during the dry summer, which negatively affects microbial abundance and activity. Compared to altered temperature, precipitation showed minor effects because it was manipulated only three months per year. Thus, the adaptative response of soil microorganisms to increased drought stress during summer may require more time.
Soil temperature is affected indirectly or directly by several factors such as solar radiation, air temperature, topography, soil water content, soil texture, and plant cover Climate change adaptation through soil and crop management: synthesis and ways forward Paul , 2004. As stated in previous studies Ćirić , 2017Dalias , 2001Sanderman , 2003, the increase of soil temperature directly influences ecosystem functioning and soil microbial processes linked to soil respiration and SOM decomposition, by altering soil processes. However, the response of soil properties and functioning to altered temperature (warming) is still uncertain and generally unknown.
Investigating the interactions between main climate drivers (e.g., changes in temperature and precipitation) and increase of atmospheric CO2 concentration is crucial to understand the potential feedbacks between future CO2 concentration and soil properties. Generally, changes of temperature (warming) and precipitation (summer drought) combined with increase of atmospheric CO2 concentration are considered one of the most important pressure factors that affects soil biodiversity Gardi , 2008, SOC concentration, and turnover rate Hillel & Rosenzweig, 2011. However, their effects are rarely additive as stated by Dieleman (2012) and Larsen (2011). Moreover, variation of temperature and precipitation combined with soil types makes the response of soils to climate drivers difficult to predict Ćirić , 2017. Dietzen (2019) investigated the effects of the increase of atmospheric CO2 concentration combined with the changes of temperature (warming) and precipitation (extended drought) on soil water variation and SOC stocks to 30 cm depth over an experimental period of 8 years. They focused particularly on determining the effect of enhanced CO2 on soil C stocks and how its effect may be altered by simultaneous changes in temperature (warming) or soil water (drought).
Conclusion¶
This synthesis resumes the state of the art of knowledge and knowledge gaps on impacts of changing climatic drivers on soil properties and functioning and adaptation of soil variables to climate change. The report provides a perspective on how individual and combined effects of climate drivers (decreased and/or increased temperature and precipitation) and enhanced CO2 concentration affect soil functioning and belowground processes as well as the responses of soil to such changes. This report was written by CREA (Italy) in collaboration with EV-ILVO (Flanders, Belgium) and SLU (Sweden), via specific search criteria (e.g., type of literature, land use, climate driver, and type of experiment) and a dedicated peer-reviewed literature analysis. A total of 39 peer-reviewed literature among original papers, synthesis and report, and meta-analysis were selected, and 41 literature items were analysed (Table 7), since two studies described the effects of climate drivers on soil processes and functioning in more than one experimental category.
Human-induced climate change is expected to continue altering climate drivers (e.g., air temperature and precipitation) and enhancing CO2 concentration in the atmosphere, also in the near-future. Changes in these conditions will alter soil processes and affect soil physical (e.g., water availability), chemical (e.g., SOM) and biological (e.g., microbial community and enzyme activity) functioning, which are of critical importance for the agricultural sector in terms of plant development and food production.
Limitation of this synthesis¶
Although this synthesis provides a clear overview of the current knowledge on the adaptative response of key soil properties and functioning to climate change based on field approaches (e.g., manipulation experiments and SFT) mainly adopted under semi-natural ecosystems, some limitation occur. Firstly, to maximize the geographic and cropland coverage of the studies, we only focused on digital peer-reviewed scientific articles written in English language, leaving out scientific articles published in paper format with no current online access and other publications such as books, book chapters, and grey literature. The restriction used for the search criteria may have limited the number of studies because no other type of documents written in English or other languages of the CLIMASOMA project consortium was included in the search criteria. Thus, relevant studied for some European environmental zones may not be considered. Secondly, the set of the selected peer-reviewed literature that fitted with our search criteria was unbalanced towards semi-natural ecosystems (e.g., grassland or mixed heterogeneous areas) in Continental, Atlantic, and Boreal environmental zones, compared to cropland in the same or in other pedoclimatic regions, such as the Mediterranean basin. Therefore, this synthesis did not provide a comprehensive set of experimental findings useful to predict the adaptative response of European agricultural soils to near-future climate change.
Recommendation and implication for future research¶
This synthesis has advanced our understanding on the impacts of climate drivers and enhanced CO2 concentration on soil properties and functioning of few specific land use and systems. Interestingly, the variables receiving the greatest attention are generally the easier-to-measure. For instance, responses of soil variables such as SOC change, Rs, MBC, MBN, and enzyme activity have received more attention (e.g., Abbasi (2020); Dieleman (2012); Wang (2016); Yu (2018)), while responses of soil physical variables more related to aggregates, water infiltration, and storage have been less investigated (e.g., Beier (2012); Caplan (2019); Groh (2020)). Therefore, predicting the individual and combined effects of climate change in different environmental zones, land use and soil management is of crucial importance to help us understand the complex mechanisms underlying the responses of soil system to climate change that are still not completely known. For instance, what are the most important mechanisms behind soil changes, and how quickly will total soil properties and functioning respond? To fill these knowledge gaps and provide scientific recommendations for national and international soil policy, the use of robust datasets from existing research on the response of soil to individual or combined climate drivers must be strengthen by new research on cropland, covering the most important European agro-environmental zones and soil type, also by promoting a better integration among observational, experimental, and modelling techniques that investigate single- and multi-factor effects. This aspect has been particularly stressed in a recent commentary published on Global Change Biology specifically addressed to advance the knowledge of the effects of climate change on different ecosystems Hanson & Walker, 2020.
- Wu, Z., Dijkstra, P., Koch, G. W., Peñuelas, J., & Hungate, B. A. (2011). Responses of terrestrial ecosystems to temperature and precipitation change: A meta-analysis of experimental manipulation. Global Change Biology, 17(2), 927–942. 10.1111/j.1365-2486.2010.02302.x
- Kreyling, J., Arfin Khan, M. A. S., Sultana, F., Babel, W., Beierkuhnlein, C., Foken, T., Walter, J., & Jentsch, A. (2017). Drought Effects in Climate Change Manipulation Experiments: Quantifying the Influence of Ambient Weather Conditions and Rain-out Shelter Artifacts. Ecosystems, 20(2), 301–315. 10.1007/s10021-016-0025-8
- Gelybó, G., Tóth, E., Farkas, C., Horel, Á., Kása, I., & Bakacsi, Z. (2018). Potential impacts of climate change on soil properties. Agrokémia És Talajtan, 67(1), 121–141. 10.1556/0088.2018.67.1.9
- Tubiello, F. N., Soussana, J.-F., & Howden, S. M. (2007). Crop and pasture response to climate change. Proceedings of the National Academy of Sciences, 104(50), 19686–19690.
- Tóth, G. (2008). Soil quality in the European Union. Threats to Soil Quality in Europe, 11–19.